Reading the rules of gene regulation
We have barely begun to crack open the rulebook for the vast noncoding regions of the genome. Two new methods, building on CRISPR advances, may help reveal some of the pages.
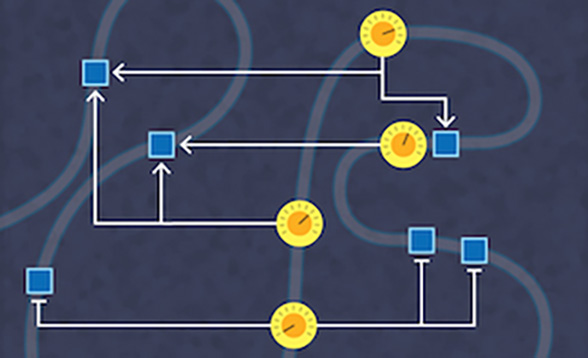
We have a reasonable understanding of the rules behind the genome’s protein-coding components. We can look at a DNA sequence and point with confidence to where a gene’s coding region begins, where it ends, and pieces of its geography.
For the remaining 98 percent of the genome — the part that dictates which genes a cell reads — it’s a different story. What knowledge we have of the rules governing this “dark matter” comes from from studying and manipulating individual bits of noncoding DNA one at a time. The rulebook that governs how the noncoding genome works, however, has remained out of reach.
“Ninety percent of the genetic variations that affect human disease are in the noncoding regions,” said Broad founding director Eric Lander. “But we haven’t had any way to tell, in a systematic way, which regulators affect which genes.”
In a pair of newly published Science papers, two research teams at the Broad show how methods leveraging CRISPR gene editing could help grasp those rules.
Using two complementary approaches, the teams — one from the Lander lab, the other from that of Broad Core Institute Member and McGovern Institute for Brain Research investigator Feng Zhang — used CRISPR as a tool to systematically probe thousands of noncoding DNA sequences simultaneously (much as Zhang and others did previously with coding DNA). In the process, both identified several interesting genetic regulators, including ones millions of bases away from the genes they control.
“We’d like to be able to catalog the noncoding elements that control every gene’s expression in every cell type,” said Jesse Engreitz, a postdoctoral fellow in the Lander lab and senior author on one of the papers. “This is a massive problem in biology, and it’s a rate-limiting step for connecting many genetic associations to their fundamental molecular mechanisms and to human disease.”
Variations on a theme
Both teams used pooled CRISPR screens (which scan and edit large swaths of the genome simultaneously using a molecular scalpel called the Cas9 enzyme and thousands of guide RNAs, which target Cas9 to specific sequences) to perturb noncoding DNA. But they did so in different ways.
Zhang, Neville Sanjana (a Zhang lab alum and now a core member of the New York Genome Center), and Jason Wright (another Zhang alum, now at Homology Medicines) used Cas9 to make precise edits to overlapping stretches of noncoding DNA — in their case, in regions surrounding three genes (NF1, NF2, and CUL3) whose functional loss has been linked to drug resistance in a form of melanoma.
“This approach lets us induce a wide diversity of mutations,” Sanjana explained. “We don’t have to speculate how a given sequence might best be disrupted.”
Engreitz, Lander, and graduate student Charles Fulco, on the other hand, employed a CRISPR interference system, using an inactive or “dead” form of Cas9 fused to a protein fragment called a KRAB domain to silence their target sequences (around MYC and GATA1, the genes for two important transcription factors).
“This system provides a good quantitative estimate of a given noncoding region’s regulatory influence,” Engreitz said. “It both shows you where the dials are that control a given gene, and tells you how much each dial matters.”
Each team then used a functional readout (increased drug resistance in melanoma cells for Sanjana, Wright, and Zhang; a drop in cell growth for Fulco, Lander, and Engreitz) and deep sequencing to see which of their guide RNAs impacted expression of their genes of interest and map the regulators those guide RNAs affected.
The two teams’ findings, confirmed with an array of additional techniques (e.g., chromatin profiling, 3D conformational capture, transcription factor profiling), point to the potential for tracing the noncoding genome’s regulatory wiring leveraging CRISPR tools. Fulco, Lander, and Engreitz found and ranked the relative importance of seven MYC and three GATA1 enhancers (short pieces of noncoding DNA that boost a gene’s chances of being read). Sanjana, Wright, and Zhang’s screen pinpointed numerous enhancers and transcription factor binding sites just for CUL3 alone.
Studying sequences in their natural habitat
While similar in principle to traditional reporter assays (where scientists couple interesting sequences to reporter genes in plasmids), these pooled CRISPR screens have a distinct difference: they probe the sequences directly, in their native habitat.
“The screens interrogate the sequences in their endogenous context,” Sanjana emphasized. “Reporter assays can be very helpful, but they lack the 3D conformation or local chromatin environment of the native genomic context. Here, the regulatory sequences undergo all of their normal interactions.”
“For example, we could see long-range loops between gene promoters and noncoding sites thousands of bases away,” he continued. “We would have missed these interesting 3D interactions entirely if we just looked at these regulatory elements in isolation.”
One limitation, Engreitz noted, is that neither CRISPR approach, in its current form, addresses the genome’s inherent redundancy. “Maybe it’s not enough to break one enhancer to really understand how a gene is controlled. Maybe you have to break more than one,” he said. “We can’t do that yet.”
But Engreitz, Sanjana, and Lander are all optimistic about the potential for using CRISPR-based approaches to reveal the noncoding genome’s underlying order.
“One interesting challenge with the noncoding genome is that while it is huge, the individual functional elements within it can be quite small,” Sanjana said. “In the future, it will be important to think about how we can develop new approaches that interrogate larger regions while maintaining high resolution.”
Engreitz agreed, adding, “There’s a potential that as we map more of these connections we’re going to learn the rules that let us predict them for the rest of the noncoding genome.”
“These approaches, using libraries of guide RNAs to bring CRISPR in to cut or bring in inhibitors, let you directly see the effects of large areas of noncoding DNA on different genes,” Lander said. “I think this is going to crack open systematic maps of gene regulation.”
Papers cited:
Fulco CP et al. Systematic mapping of functional enhancer-promoter connections with CRISPR interference. Science. September 29, 2016. DOI: 10.1126/science.aag2445
Sanjana NE et al. High-resolution interrogation of functional elements in the noncoding genome. Science. September 29, 2016. DOI: 10.1126/science.aaf7613