Squishy, stealthy neural probes
New implantable devices transform and soften to match the mechanical properties of the brain, enabling long-term studies of neural circuits.
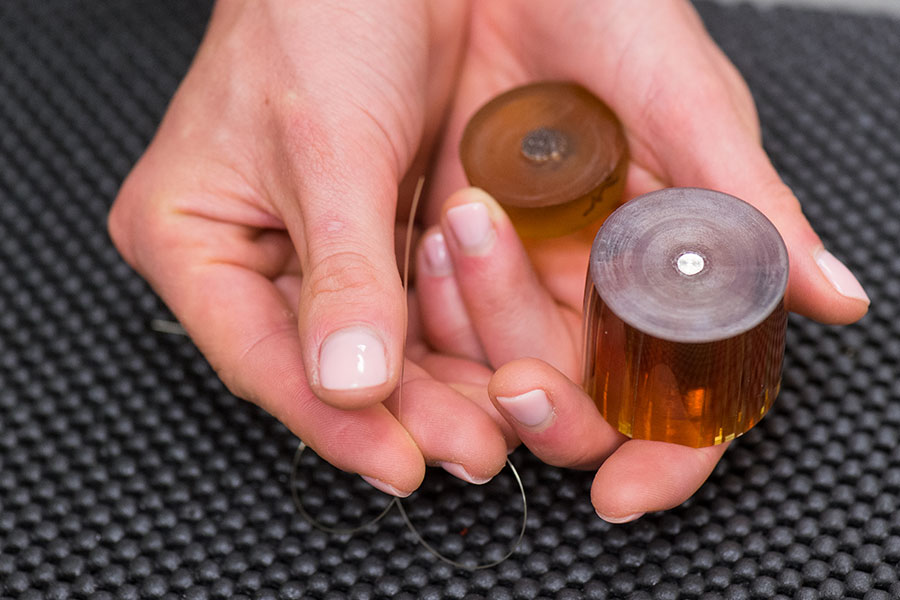
Slender probes equipped with electrodes, optical channels, and other tools are widely used by neuroscientists to monitor and manipulate brain activity in animal studies. Now, scientists at MIT have devised a way to make these usually rigid devices become as soft and pliable as their surroundings when they are implanted in the brain. Their new multifunctional devices are less intrusive than traditional neuroscience probes and remain functional for months after implantation, enabling long-term studies of neural circuits in animal models.
Researchers led by McGovern Institute scientist Polina Anikeeva built the new devices by embedding their functional components in a water-absorbing hydrogel. Each device begins as stiff probe able to penetrate brain tissue. But once it is in place, the hydrogel absorbs water and the device transforms.
“When it’s dry, it’s completely rigid. Its mechanics are dominated by mechanics of the polymers and metals that went into it,” explains Anikeeva, who is also an associate professor in the Departments of Materials Science and Engineering and Brain and Cognitive Sciences. “When it’s fully hydrated, it has the [mechanical] properties of the brain.”
Anikeeva and colleagues reported on the new devices in the June 8 issue of Nature Communications.
Stealthy probes
Neural probes made out of metal or hard plastics have been invaluable in neuroscience research, allowing scientists to sense electrical activity within the brain, supply drugs to specific locations, or deliver neuron-activating pulses of light.
In 2015, Anikeeva and her group developed multifunctional probes, which are equipped with the tools to do all of these things. Although these polymer based devices were more biocompatible than metals and semiconductors, which can cut like tiny knives through the soft, jiggly tissue of the brain, their mechanics were still orders of magnitude away from those of neural tissue. Most neural probes can be used for a few weeks, until scar tissue forms around them and interferes with their function.
“For some experiments, this may not matter,” Anikeeva says. “But for other experiments, it does. If, for example, you’re interested in how a neuron evolves over the course of long-term behavior, or aging, or development, it’s important to keep track of the same tissue or the same cells. And that was challenging [with rigid probes].”
To enable longer experiments, Anikeeva’s team began to think about making multifunctional probes out of a material that is more compatible with the brain. “We wanted to create a device that would be stealthy, so the brain wouldn’t know that it’s there,” she says. To be useful, the device would still need some amount of hard material. But electrodes, microfluidic chambers, and optical channels can be tiny—just a fraction of the width of a human hair. “Even if they’re made out of polymer or soft metal, if you make them that small, they become sufficiently soft that they will be able to move with the brain and not cause damage,” Anikeeva says. It is the polymer matrix that surrounds these functional components that gives neural probes their shape and rigidity, which despite causing problems once inside the brain, is essential for implantation.
Seongjun Park, a graduate student in Anikeeva’s group, and Hyunwoo Yuk, another MIT graduate student who had been working with hydrogels in Xuanhe Zhao’s mechanical engineering lab, discussed the problem and proposed a probe that took advantage of that material. Because of hydrogels’ tunable nature, they could be used to build a device that was both stealthily squishy and piercingly rigid. By fine-tuning the chemistry, the team could ensure that after the device was implanted, its hydrogel would absorb just enough water to closely match the mechanics of the brain.
Hydrogel glue
Other researchers had previously developed neural probes wrapped in a hydrogel covering, but Anikeeva’s team wanted the hydrogel to be the bulk of the device. They would use the swellable material to bundle together the functional elements and fill the space between them.
To do so, they assembled the fibers that would give their device its desired function—an electrode array fiber for sensing neural activity, an optical fiber for delivering light to manipulate signaling, and a fluidic fiber for delivering drugs and genes—and chemically treated them so that they would adhere directly to the components of a hydrogel.
They then dipped the treated fibers into a solution of a hydrogel-forming compound called alginate. By exposing the solution to light, they triggered the alginate to polymerize, ultimately creating a thin strand of the hydrogel with the functional fibers embedded within it.
When it is first pulled out of the solution, Anikeeva says, the hydrogel-based device is like a wet noodle, with its components moving freely within it like the bendable bristles of a wet paintbrush. As the hydrogel dries, the fibers become firmly affixed to one another and the entire device stiffens—much like a drying paintbrush.
Long-term tracking
To test the devices, Anikeeva’s team implanted them into mice, targeting anxiety circuits deep within the brain. They behaved exactly as they had hoped—easily penetrating into the tissue, then returning to their “wet noodle” state and remaining in place without triggering a foreign body response in the brain. After more than six months of recording neural activity, the probes remained fully functional.
Anikeeva says her team’s squishy new probes are the first multifunctional neural devices to remain effective in living animals for this prolonged period. The improved longevity of the devices compared to their predecessors means researchers will be able to use them to track and manipulate neuronal behavior during long-term processes such as learning, disease progression, and aging.
The team is already working on the next-generation of hydrogel probes, which will further take advantage of the material’s unique properties to control the release of drugs or other compounds within the brain and improve the devices’ biocompatibility. And with a simplified fabrication process in development, Anikeeva says it may soon be possible for neuroscientists to manufacture the stealthy probes in their own labs.