Mapping the cellular circuits behind spitting
Roundworms change the flow of material in and out of their mouths in response to bright light, revealing a new way for neurons to control muscle cells.
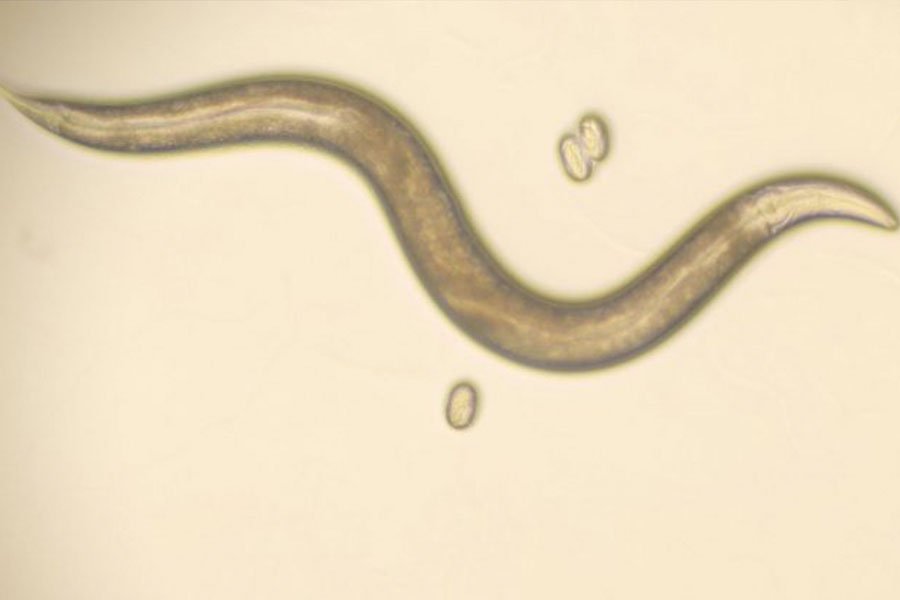
Now, in a study recently published in eLife, a team led by former graduate student Steve Sando reports the mechanism that underlies spitting in C. elegans. Individual muscle cells are generally regarded as the smallest units that neurons can independently control, but the researchers’ findings question this assumption. In the case of spitting, they determined that neurons can direct specialized subregions of a single muscle cell to generate multiple motions — expanding our understanding of how neurons control muscle cells to shape behavior.
“Steve made the remarkable discovery that the contraction of a small region of a particular muscle cell can be uncoupled from the contraction of the rest of the same cell,” says H. Robert Horvitz, the David H. Koch Professor of Biology at MIT, a member of the McGovern Institute for Brain Research and the Koch Institute for Integrative Cancer Research, Howard Hughes Medical Institute Investigator, and senior author of the study. “Furthermore, Steve found that such subcellular muscle compartments can be controlled by neurons to dramatically alter behavior.”
Roundworms are like vacuum cleaners that wiggle around hoovering up bacteria. The worm’s mouth, also known as the pharynx, is a muscular tube that traps the food, chews it, and then transfers it to the intestines through a series of “pumping” contractions.
Researchers have known for over a decade that worms flee from UV, violet, or blue light. But Bhatla discovered that this light also interrupts the constant pumping of the pharynx, because the taste produced by the light is so nasty that the worms pause feeding. As he looked closer, Bhatla noticed the worms’ response was actually quite nuanced. After an initial pause, the pharynx briefly starts pumping again in short bursts before fully stopping — almost like the worm was chewing for a bit even after tasting the unsavory light. Sometimes, a bubble would escape from the mouth, like a burp.
After he joined the project, Sando discovered that the worms were neither burping nor continuing to munch. Instead, the “burst pumps” were driving material in the opposite direction, out of the mouth into the local environment, rather than further back into the pharynx and intestine. In other words, the bad-tasting light caused worms to spit. Sando then spent years chasing his subjects around the microscope with a bright light and recording their actions in slow motion, in order to pinpoint the neural circuitry and muscle motions required for this behavior.
“The discovery that the worms were spitting was quite surprising to us, because the mouth seemed to be moving just like it does when it’s chewing,” Sando says. “It turns out that you really needed to zoom in and slow things down to see what’s going on, because the animals are so small and the behavior is happening so quickly.”
To analyze what’s happening in the pharynx to produce this spitting motion, the researchers used a tiny laser beam to surgically remove individual nerve and muscle cells from the mouth and discern how that affected the worm’s behavior. They also monitored the activity of the cells in the mouth by tagging them with specially-engineered fluorescent “reporter” proteins.
They saw that while the worm is eating, three muscle cells towards the front of the pharynx called pm3s contract and relax together in synchronous pulses. But as soon as the worm tastes light, the subregions of these individual cells closest to the front of the mouth become locked in a state of contraction, opening the front of the mouth and allowing material to be propelled out. This reverses the direction of the flow of the ingested material and converts feeding into spitting.
The team determined that this “uncoupling” phenomenon is controlled by a single neuron at the back of the worm’s mouth. Called M1, this nerve cell spurs a localized influx of calcium at the front end of the pm3 muscle likely responsible for triggering the sub-cellular contractions.
M1 relays important information like a switchboard. It receives incoming signals from many different neurons, and transmits that information to the muscles involved in spitting. Sando and his team suspect that the strength of the incoming signal can tune the worm’s behavior in response to tasting light. For instance, their findings suggest that a revolting taste elicits a vigorous rinsing of the mouth, while a mildly unpleasant sensation causes the worm spit more gently, just enough to eject the contents.
In the future, Sando thinks the worm could be used as a model to study how neurons trigger subregions of muscle cells to constrict and shape behavior — a phenomenon they suspect occurs in other animals, possibly including humans.
“We’ve essentially found a new way for a neuron to move a muscle,” Sando says. “Neurons orchestrate the motions of muscles, and this could be a new tool that allows them to exert a sophisticated kind of control. That’s pretty exciting.”