When we read, information zips between language processing centers in different parts of the brain, traveling along neural highways in the white matter. This coordinated activity allows us to decipher words and comprehend their meaning. Many neuroscientists suspect that variations in white matter may underlie differences in reading ability, and hope that by determining which white matter tracts are involved, they will be able to guide the development of more effective interventions for children who struggle with reading skills.
In a January 14, 2022, online publication in the journal NeuroImage, scientists at MIT’s McGovern Institute report on the largest brain imaging study to date to evaluate the relationship between white matter structure and reading ability. Their findings suggest that if white matter deficiencies are a significant cause of reading disability, new strategies will be needed to pin them down.
White matter is composed of bundles of insulated nerve fibers. It can be thought of as the internet of the brain, says senior author John Gabrieli, the Grover Hermann Professor of Health Sciences and Technology at MIT. “It’s the connectivity: the way that the brain communicates at some distance to orchestrate higher-level thoughts, and abilities like reading,” explains Gabrieli, who is also a professor of brain and cognitive sciences and an investigator at the McGovern Institute.
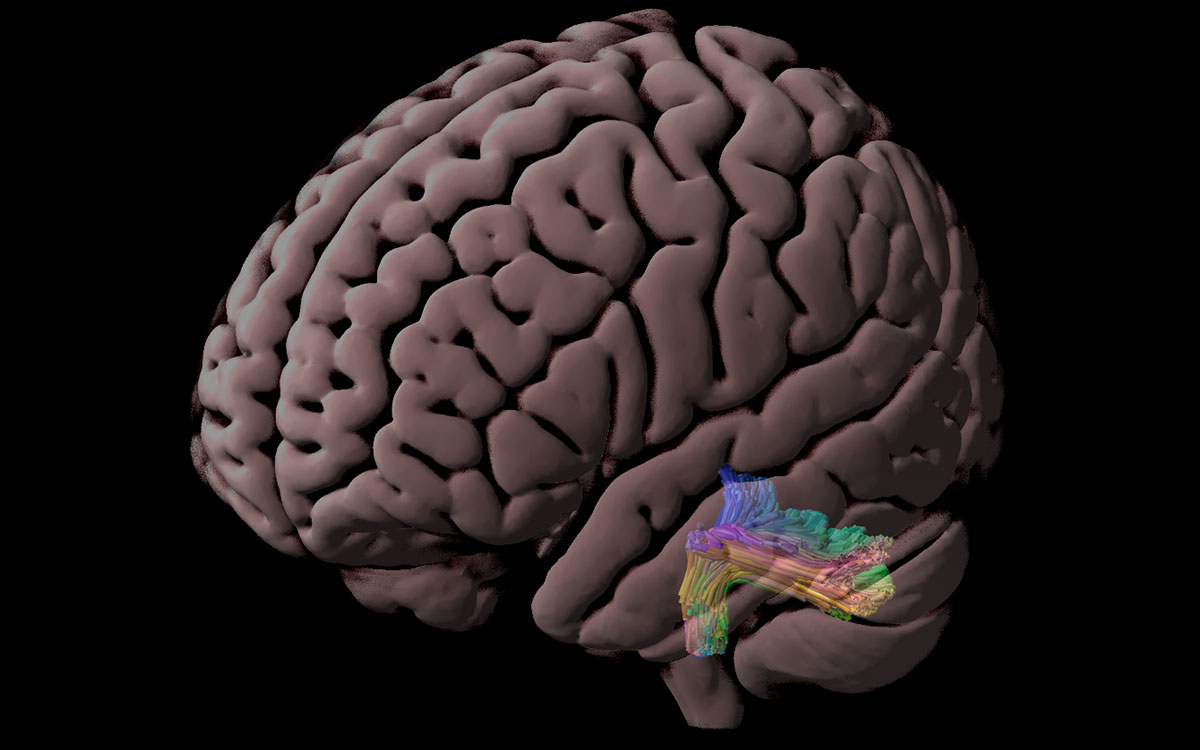
Long-distance connections
To visualize white matter and study its structure, neuroscientists use an imaging technique called diffusion-weighted imaging (DWI). Images are collected in an MRI scanner by tracking the movements of water molecules in the brain. A key measure used to interpret these images is fractional anisotropy (FA), which varies with many physical features of nerve fibers, such as their density, diameter, and degree of insulation. Although FA does not measure any of these properties directly, it is considered an indicator of structural integrity within white matter tracts.
Several studies have found the FA of one or more white matter tracts to be lower in children with low reading scores or dyslexia than in children with stronger reading abilities. But those studies are small—usually involving only a few dozen children—and their findings are inconsistent. So it has been difficult to attribute reading problems to poor connections between specific parts of the brain.
Hoping to glean more conclusive results, Gabrieli and Steven Meisler, a graduate student in the Harvard Program in Speech and Hearing Bioscience and Technology who is completing his doctoral work in the Gabrieli lab, turned to a large collection of high-quality brain images available through the Child Mind Institute’s Healthy Brain Network. Using DWI images collected from 686 children and state-of-the-art methods of analysis, they assessed the FA of 20 white matter tracts that are thought to be important for reading.
The children represented in the dataset had diverse reading abilities, but surprisingly, when they compared children with and without reading disability, Meisler and Gabrieli found no significant differences in the FA of any of the 20 tracts. Nor did they find any correlation between white matter FA and children’s overall reading scores.
More detailed analysis did link reading ability to the FA of two particular white matter tracts. The researchers only detected the correlation when they narrowed their analysis to children older than eight, who are usually reading to learn, rather than learning to read. Within this group, they found two white matter tracts whose FA was lower in children who struggled with a specific reading skill: reading “pseudowords.” The ability to read nonsense words is used to assess knowledge of the relationship between letters and sounds, since real words can be recognized instead through experience and memory.
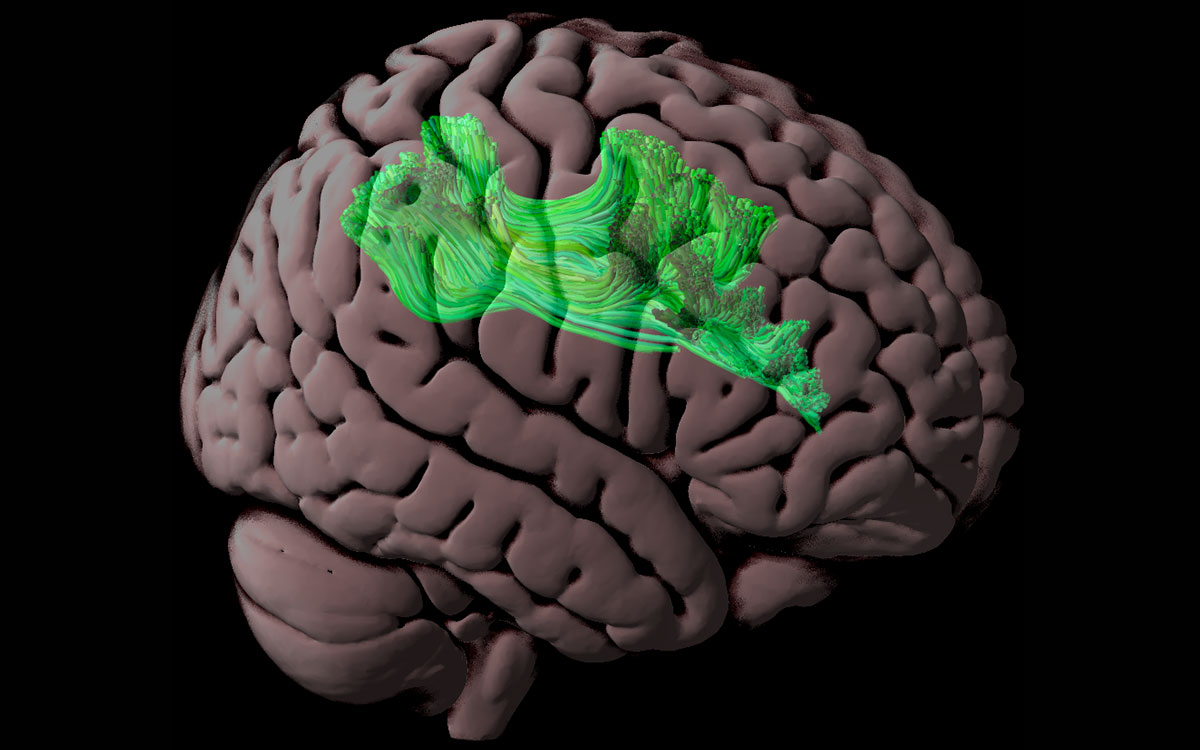
The first of these tracts connects language processing centers in the frontal and parietal brain regions. The other contains fibers that connect that the brainstem with the cerebellum, and may help control the eye movements needed to see and track words. The FA differences that Meisler and Gabrieli linked to reading scores were small, and it’s not yet clear what they mean. Since less cohesive structure in these two tracts was linked to lower pseudoword-reading scores only in older children, it may be a consequence of living with a reading disability rather than a cause, Meisler says.
The findings don’t rule out a role for white matter structure in reading disability, but they do suggest that researchers will need a different approach to find relevant features. “Our results suggest that FA does not relate to reading abilities as much as previously thought,” Meisler says. In future studies, he says, researchers will likely need to take advantage of more advanced methods of image analysis to assess features that more directly reflect white matter’s ability to serve as a conduit of information.