More information about each series in the McGovern Institute postcard collection, including the color-your-own mindfulness postcards, can be found below.
Mindfulness Postcard Series, 2023
In winter 2023, the institute released its mindfulness postcard series, a collection of four different neuroscience-themed illustrations that can be colored in with pencils, markers, or paint. The postcard series was inspired by research conducted in John Gabrieli’s lab, which found that practicing mindfulness reduced children’s stress levels and negative emotions during the pandemic. These findings contribute to a growing body of evidence that practicing mindfulness — focusing awareness on the present, typically through meditation, but also through coloring — can change patterns of brain activity associated with emotions and mental health.
Download and color your own postcards.
Genes
The McGovern Institute is at the cutting edge of applications based on CRISPR, a genome editing tool pioneered by McGovern Investigator Feng Zhang. Hidden within this DNA-themed postcard is a clam, virus, bacteriophage, snail, and the word CRISPR. Click the links to learn how these hidden elements relate to genetic engineering research at the McGovern Institute.
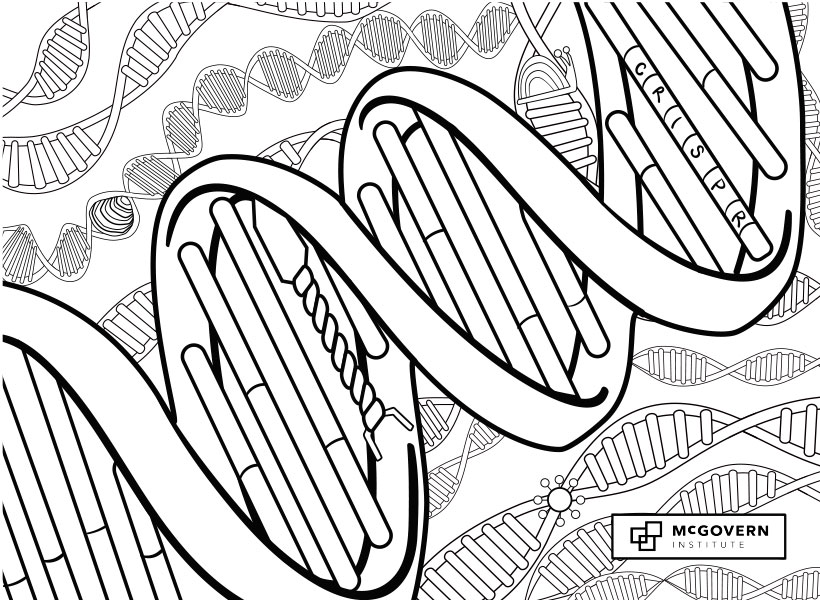
Neurons
McGovern researchers probe the nanoscale and cellular processes that are critical to brain function, including the complex computations conducted in neurons, to the synapses and neurotransmitters that facilitate messaging between cells. Find the mouse, worm, and microscope — three critical elements related to cellular and molecular neuroscience research at the McGovern Institute — in the postcard below.
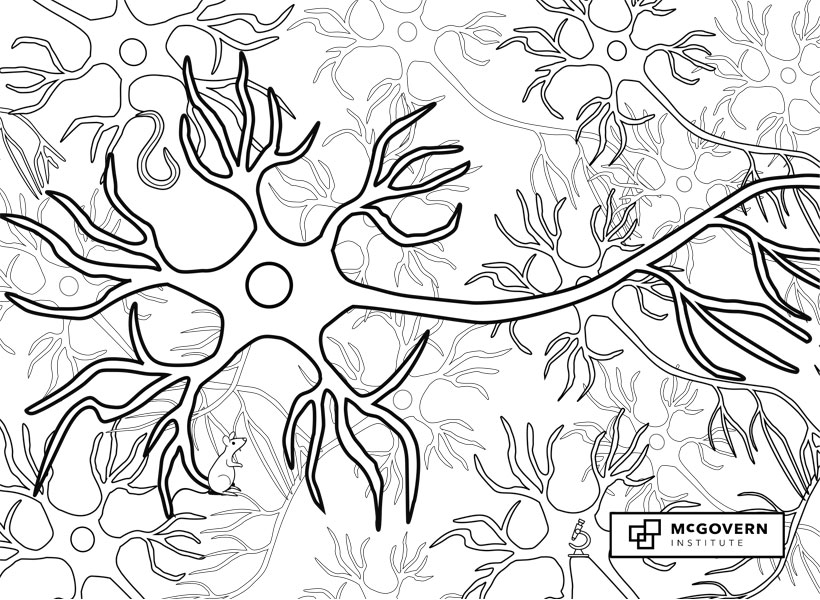
Human Brain
Cognitive neuroscientists at the McGovern Institute examine the brain processes that come together to inform our thoughts and understanding of the world. Find the musical note, speech bubbles, and human face in this postcard and click on the links to learn more about how these hidden elements relate to brain research at the McGovern Institute.
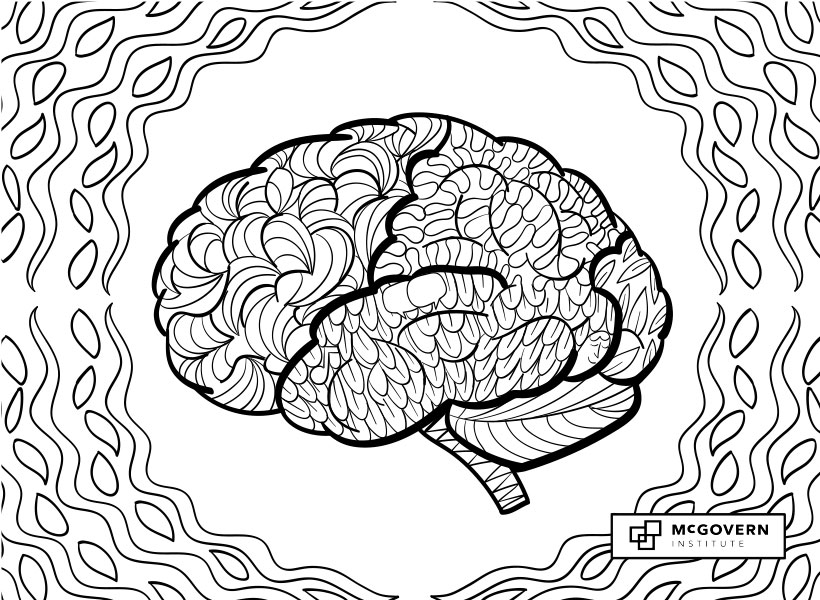
Artificial Intelligence
McGovern researchers develop machine learning systems that mimic human processing of visual and auditory cues and construct algorithms to help us understand the complex computations made by the brain. Find the speech bubbles, DNA, and cochlea (spiral) in this postcard and click on the links to learn more about how these hidden elements relate to computational neuroscience research at the McGovern Institute.
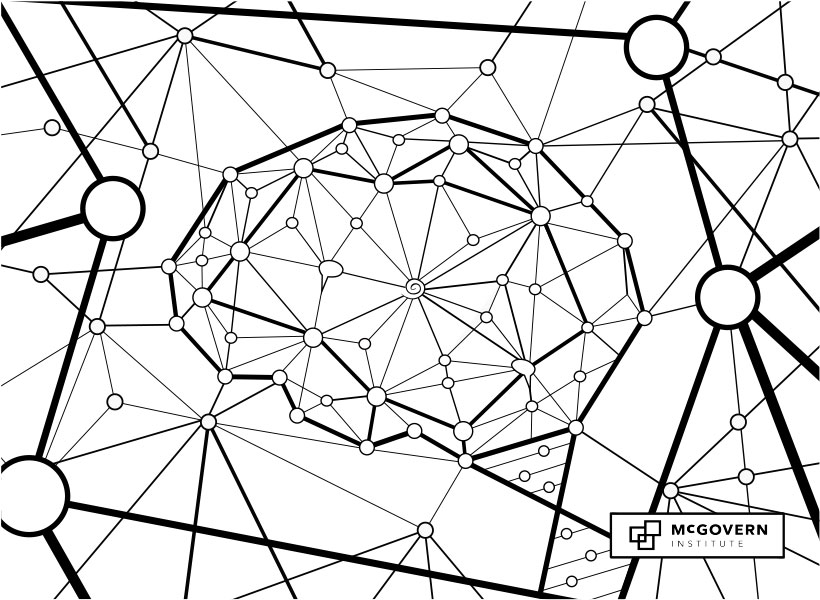
Neuron Postcard Series, 2019
In 2019, the McGovern Institute released a second series of postcards based on the anatomy of a neuron. Each postcard includes text on the back side that describes McGovern research related to that specific part of the neuron. The descriptive text for each postcard is shown beloSynapse
Synapse
Signals flow through the nervous system from one neuron to the next across synapses.
Synapses are exquisitely organized molecular machines that control the transmission of information.
McGovern researchers are studying how disruptions in synapse function can lead to brain disorders like autism.
Image: Joseph Laney
Axon
The axon is the long, thin neural cable that carries electrical impulses called action potentials from the soma to synaptic terminals at downstream neurons.
Researchers at the McGovern Institute are developing and using tracers that label axons to reveal the elaborate circuit architecture of the brain.
Image: Joseph Laney
Soma
The soma, or cell body, is the control center of the neuron, where the nucleus is located.
It connects the dendrites to the axon, which sends information to other neurons.
At the McGovern Institute, neuroscientists are targeting the soma with proteins that can activate single neurons and map connections in the brain.
Image: Joseph Laney
Dendrites
Long branching neuronal processes called dendrites receive synaptic inputs from thousands of other neurons and carry those signals to the cell body.
McGovern neuroscientists have discovered that human dendrites have different electrical properties from those of other species, which may contribute to the enhanced computing power of the human brain.
Image: Joseph Laney
Brain Anatomy Postcard Series, 2017
The original brain anatomy-themed postcard series, developed in 2017, was inspired by the U.S. Works Projects Administration’s iconic national parks posters created in the 1930s and 1940s. Each postcard includes text on the back side that describes McGovern research related to that specific part of the neuron. The descriptive text for each postcard is shown below.
Sylvian Fissure
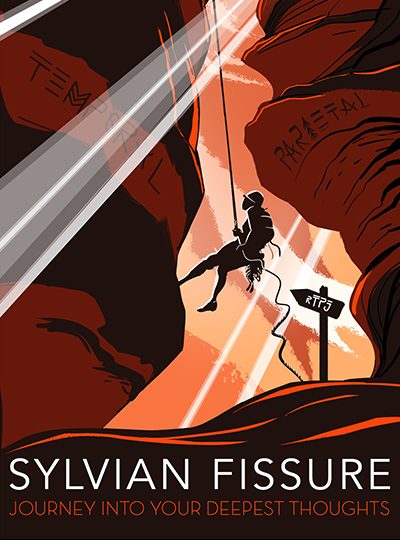
Hippocampus
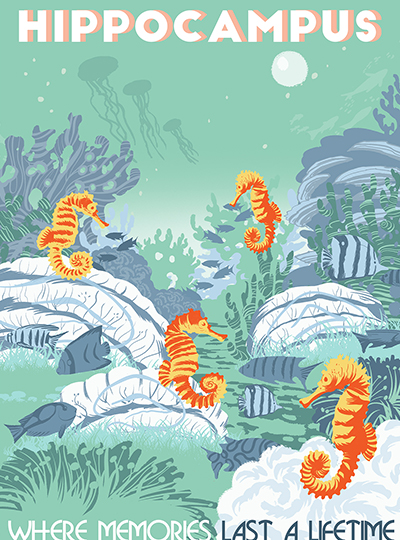
Basal Ganglia
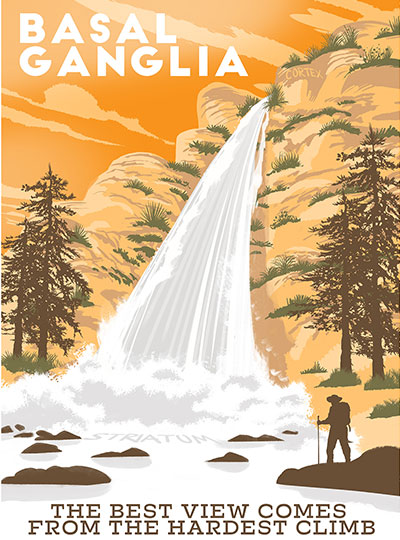
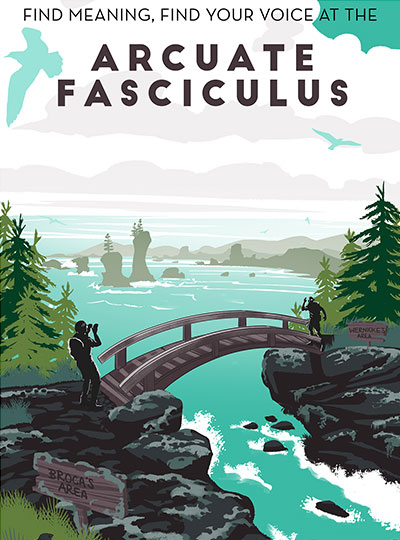
Order and Share
To order your own McGovern brain postcards, contact our colleagues at the MIT Museum, where proceeds will support current and future exhibitions at the growing museum.
Please share a photo of yourself in your own lab (or natural habitat) with one of our cards on social media. Tell us what you’re studying and don’t forget to tag us @mcgovernmit using the hashtag #McGovernPostcards.